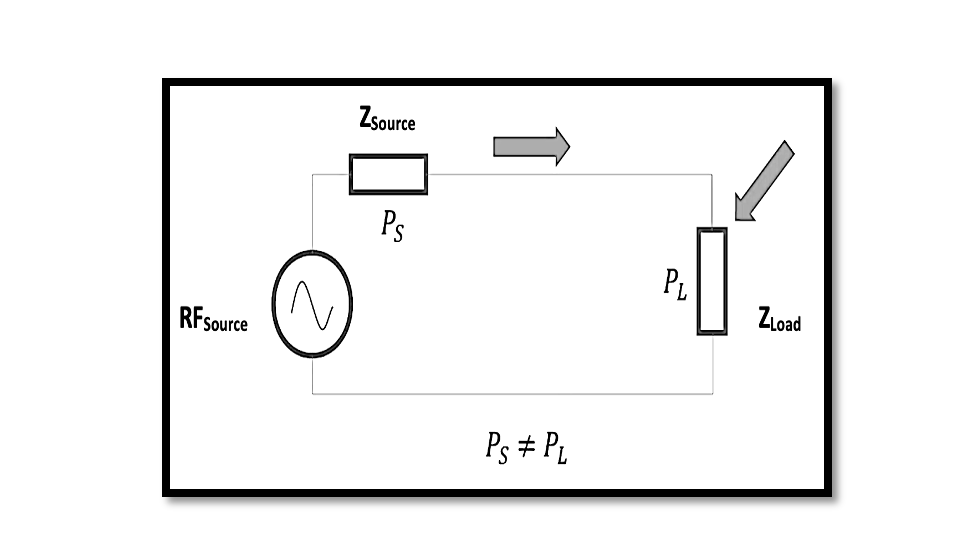
Understanding RF Signal Transmission Loss in Factory Testing
Introduction
RF losses in signal transmission play a pivotal role in the efficacy of factory testing and the integrity of RF circuits. Understanding these losses, their causes, and how to measure them is crucial for optimizing performance. Let’s delve into the world of RF losses and their impact on signal transmission.
RF power loss occurs during the propagation of an RF signal due to various factors such as impedance mismatch, dielectric loss, and conductor loss. In an ideal scenario, termed the ‘lossless case,’ there would be no losses, and all power would transfer seamlessly from the source to the load. However, real-world scenarios involve imperfections leading to signal degradation. Factory testing involving test scripts implemented with RF signals faces a critical consideration: RF losses. These losses significantly impact the effectiveness of the testing process. In this comprehensive guide, we’ll delve into the realm of RF losses, understanding their implications on factory testing, and how to effectively measure them. Exploring both theoretical and practical aspects, we’ll navigate through ideal and real scenarios of RF signal transmission, methods to measure losses, and crucial techniques to manage RF losses within factory setups.
Measuring and Managing RF Signal Transmission Losses
- Calculation and Estimation
Accurate prediction of losses allows for informed adjustments in setup configurations. Calculation or estimation methodologies aid in anticipating losses, enabling proactive measures to optimize power delivery to the load.
- De-embedding of Power
The concept of de-embedding power focuses on understanding and compensating for losses in signal magnitude. By disentangling power losses within the setup, this technique assists in enhancing the efficiency of power transfer.
- Power Correlation Techniques
Implementing power correlation techniques enables meticulous tracking and efficient management of RF losses within the factory testing setup. This systematic approach helps in maintaining an optimal power flow, crucial for accurate testing outcomes.
RF Signal Transmission Loss: Theoretical Overview (Lossless Case)
In the scenario of a lossless case, an ideal condition prevails where the system experiences no loss, ensuring the entirety of power transference from the source to the load. Here’s what defines the lossless case:
1.1 Uniform Impedance: Maximum power transfer occurs when impedances across the circuit, including the source, transmission line, and load, match perfectly. This alignment ensures optimal power transfer to the load.
1.2 Absence of Parasitic Effects: Any absence of capacitance, inductance, or resistive components within the circuit avoids alterations in behavior across frequencies. This absence prevents dissipation that could result in power loss before reaching the load.
The uniformity of impedance across all components is pivotal. According to the maximum power transfer theorem, optimal power transfer occurs when the impedance across each segment of the circuit, including the source, transmission line, and load, aligns perfectly. This alignment ensures an unhindered flow of power throughout the circuit, reaching its destination, the load, in its entirety.
Moreover, the absence of parasitic effects plays a crucial role. In this context, the absence of capacitance, inductance, or any resistive elements within the sources or transmission lines is imperative. Such absence guarantees a consistent behavior of the circuit across varying frequencies, preventing any dissipation that might lead to power loss before reaching the intended load.
Real-World Scenario: Understanding Losses (Lossy Case)
Lossy Case Real-world scenarios realistic behavior of a transmission line, subject to various contributors that impact signal losses: impedance mismatch, dielectric loss, and conductor loss:
2.1 Impedance Mismatch: Deviations from uniform impedance, even slight differences, cause reflections. Mismatches between the source, transmission line, and load lead to reflections and a portion of RF power being sent back to the source.
Impedance uniformity across the circuit, as emphasized by the maximum power transfer theorem, is crucial. Even slight discrepancies between the impedance of the source, transmission line, and load trigger reflections, diverting a fraction of RF power back to the source. These discrepancies, although often minimal – for instance, 50 ohms may vary slightly to 51 or 49 ohms – result in reflections, particularly pronounced when the characteristic impedance deviates significantly from the source or load impedance. Such deviations cause reflected waves at connection points, hindering complete power transfer to the load.
2.2 Parasitic Effects: Inductance and capacitance within components cause impedance shifts with frequency changes, contributing to signal drop-offs, particularly at transition points along the transmission line.
Parasitic effects stemming from inductance and capacitance within components further aggravate impedance mismatch. Frequency-induced alterations in impedance, caused by series inductance and capacitance to ground, lead to signal drop-offs along the transmission line. These parasitic effects introduce additional impedances, impacting the overall flow of RF power.
2.3 Dielectric and Conductor Losses: Dielectric loss occurs as the dielectric material absorbs RF power, akin to a resistor parallel to the load. Conductor losses, attributed to the inherent resistance of the transmission line material, result in dissipation of power as heat.
Dielectric loss emerges from the absorption of RF power, akin to a resistor in parallel with the load. This absorption results in dissipation, reducing the effective power available to the load. Conductor losses, attributed to the inherent resistance of transmission line materials, cause dissipation of power as heat. Although minute, the resistance across the transmission line contributes to overall dissipation, evident when measuring with a multimeter.
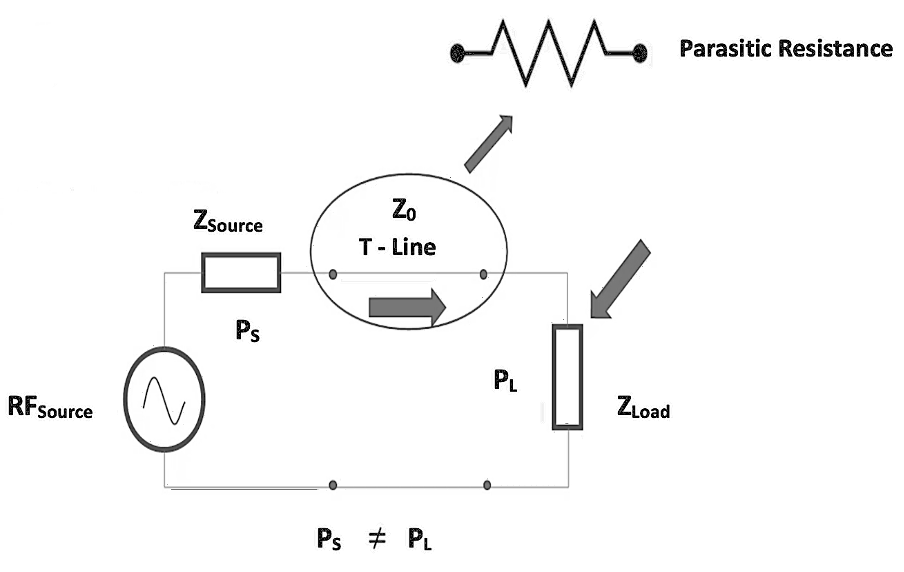
In summary, the lossy case presents a comprehensive picture of real-world transmission line behavior, affected by impedance mismatch, parasitic impedance, dielectric, and conductor losses. These factors collectively lead to signal attenuation and dissipation, crucial considerations in optimizing RF signal transmission within factory testing setups.
Example
Let’s illustrate an example using a transmission line with a 1dB loss. Assuming the impedance of the source, load, and transmission line are all set to 50 ohms, the 1dB loss might stem from conductor or dielectric losses, common in practical scenarios. It’s worth noting that a 1dB loss might be considered tolerable in certain transmission line lengths. The objective here is to calculate the power received at the load when setting the RF source to a specific power level. In this case, the power delivered to the load equals the power set by the source minus the 1dB loss incurred in the transmission line. Essentially, the power experiences a 1dB reduction as it traverses from the source through the transmission line to the load. Therefore, at the load, the received power will be 1dB less than the power initially set at the RF source. This calculation aids in understanding the effective power reaching the load, considering the losses encountered along the transmission line.
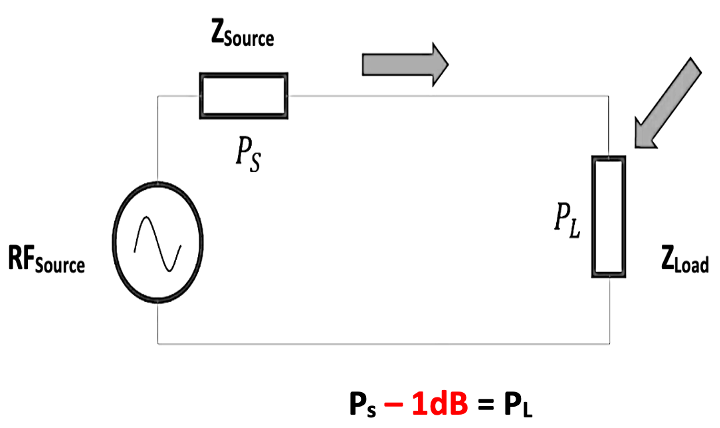
Conclusion
RF signal transmission losses significantly impact factory testing outcomes. Understanding the intricacies of lossless and lossy scenarios, measuring losses, and implementing management techniques are pivotal for optimizing testing processes. By comprehensively addressing RF losses, factory setups can enhance their efficiency and accuracy, ensuring robust testing protocols.
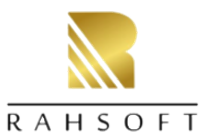
Learn more about this topic by taking the complete course ‘Introduction to RF Testing Fundamentals and RF Test Architecture – RAHRF412’. Watch the course videos for more detailed understanding. Also checkout other courses on RF system and IC design on https://rahsoft.com/courses/. Rahsoft also provides a certificate on Radio Frequency. All the courses offer step by step approach.